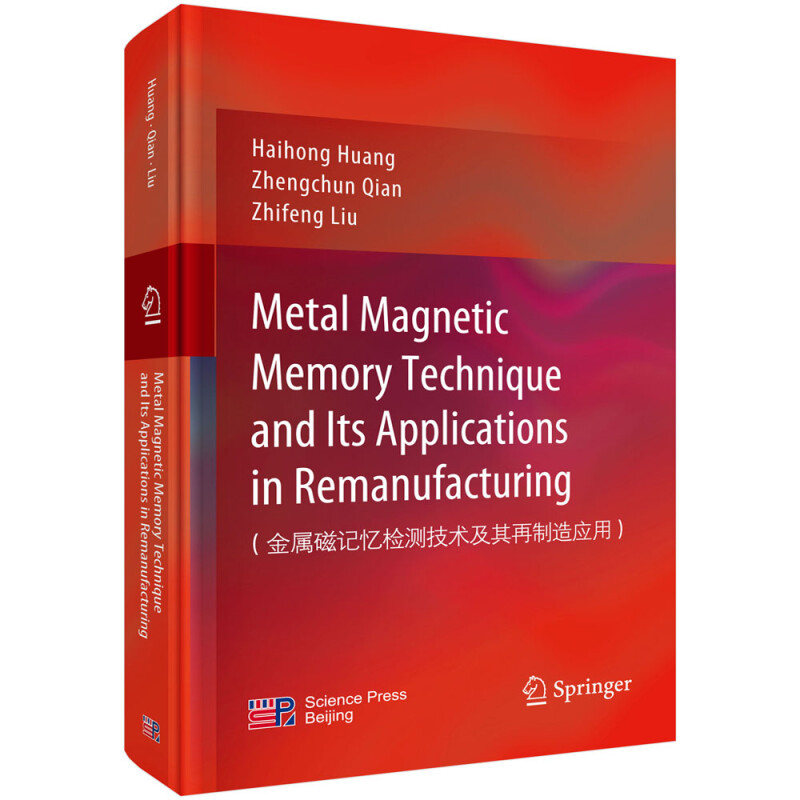
包邮金属磁记忆检测技术及其再制造应用(英文版)

- ISBN:9787030702487
- 装帧:一般胶版纸
- 册数:暂无
- 重量:暂无
- 开本:16开
- 页数:240
- 出版时间:2021-01-01
- 条形码:9787030702487 ; 978-7-03-070248-7
内容简介
本书旨在将一种新型的无损检测方法,即金属磁记忆技术引入到再制造工程领域,为再制造产品的质量控制提供指导。书中详细介绍了金属磁记忆的研究现状及其在再制造中的应用前景,阐述了如何利用磁记忆信号检测再制造之前毛坯的损伤程度,分析了不同载荷形式和环境变化对检测结果的影响;并且对再制造之后零部件修复质量开展了系统评价,建立了磁记忆信号特征与再制造涂层及其界面性能之间的映射关系;很后根据金属磁记忆基本原理开发了一套高精度的磁信号检测设备,并将书中总结提出的磁记忆理论模型、分析方法以及检测结果推广到汽车驱动桥壳、液压缸、发动机曲轴等零部件的实际再制造过程中,为金属磁记忆在再制造工程中的应用奠定坚实基础。
目录
Applications for Remanufacturing Problems and Prospects
Part I Introduction to the Metal Magnetic Memory (MMM) Technique
1 Nondestructive Testing for Remanufacturing 3
1.1 Motivations 3
1.2 Conventional Nondestructive Testing Techniques 5
1.3 MMM Technique 5
1.4 Organization of This Book 7
References 8
2 Theoretical Foundation of the MMM Technique 9
2.1 Background 10
2.2 Microscopic Mechanism 13
2.3 Macroscopic Theoretical Model 13
2.3.1 Magnetomechanical Model 17
2.3.2 Magnetic Charge Model 19
2.3.3 First Principle Theory 23
References 23
3 State of the Art of the MMM Technique 25
3.1 Historical Background 25
3.2 Theoretical Research 26
3.3 Experimental Research 27
3.4 Standard Establishment 30
3.5 Applications for Remanufacturing 31
3.6 Problems and Prospects 32
References 34
Part II Detection of Damage in Ferromagnetic Remanufacturing Cores by the MMM Technique 39
4 Stress Induces MMM Signals 39
4.1 Intxoductioii 39
4.2 Variations in the MMM Signals Induced by Static Stress 40
4.2.1 Under the Elastic Stage 41
4.2.2 Under the Plastic Stage 42
4.2.3 Theoretical Analysis 44
4.3 Variations in the MMM Signals Induced by Cyclic Stress 45
4.3.1 Under Different Stress Cycle Numbers 46
4.3.2 Characterization of Fatigue Crack Propagation 49
4.4 Conclusions 52
References 52
5 Frictional Wear Induces MMM Signals 55
5.1 Introduction 55
5.2 Reciprocating Sliding Friction Damage 56
5.2.1 Variations in the Tribology Parameters During Friction 58
5.2.2 Variations in the Magnetic Memory Signals Parallel to Sliding 60
5.2.3 Variations in the Magnetic Memory Signals Normal to Sliding 62
5.2.4 Relationship Between the Tribology 65
Characteristics and Magnetic Signals 66
5.3 Single Disassembly Friction Damage 68
5.3.1 Surface Damage and Microstructure Analysis 69
5.3.2 Variations in the MMM Signals 73
5.3.3 Damage Evaluation of Disassembly 76
5.3.4 Verification for Feasibility and Repeatability 80
5.4 Conclusions 81
References 81
6 Stress Concentration Impacts on MMM Signals 83
6.1 Introduction 84
6.2 Stress Concentration Evaluation Based on the Magnetic Dipole Model 84
6.2.1 Establishment of the Magnetic Dipole Model 86
6.2.2 Characterization of the Stress Concentration Degree 86
6.2.3 Contributions of Stress and Discontinuity to MMM Signals 91
6.3 Stress Concentration Evaluation Based on the Magnetic Dual-Dipole Model 95
6.3.1 Magnetic Scalar Potential 95
6.3.2 Magnetic Dipole and Its Scalar Potential 97
6.3.3 Measurement Process and Results 100
6.3.4 Analysis of the Magnetic Scalar Potential 103
6.4 Stress Concentration Inversion Method 110
6.4.1 Inversion Model of the Stress Concentration Based on the Magnetic Source Distribution 110
6.4.2 Inversion of a One-Dimensional Stress Concentration 112
6.4.3 Inversion of a Two-Dimensional Stress Concentration 114
6.5 Conclusions 114
References 115
7 Temperature Impacts on MMM Signals 117
7.1 Introduction 117
7.2 Modified J-A Model Based on Thermal and Mechanical Effects 117
7.2.1 Effect of Static Tensile Stress on the Magnetic Field 118
7.2.2 Effect of Temperature on the Magnetic Field 119
7.2.3 Variation in the Magnetic Field Intensity 120
7.3 Measurement of MMM Signals Under Different Temperatures 121
7.3.1 Material Preparation 122
7.3.2 Testing Method 122
7.4 Variations in MMM Signals with Temperature and Stress 123
7.4.1 Normal Component of the Magnetic Signal 125
7.4.2 Mean Value of the Normal Component of the Magnetic Signal 128
7.4.3 Variation Mechanism of the Magnetic Signals Under Different Temperatures 130
7.4.4 Analysis Based on the Proposed Theoretical Model 131
7.5 Conclusions 132
References 132
8 Applied Magnetic Field Strengthens MMM Signals 133
8.1 Introduction 133
8.2 MMM Signal Strengthening Effect Under Fatigue Stress 134
8.2.1 Variations in the MMM Signals with an Applied Magnetic Field 135
8.2.2 Theoretical Explanation Based on the Magnetic Dipole Model 137
8.3 MMM Signal Strengthening Effect Under Static Stress 139
8.3.1 Magnetic Signals Excited by the Geomagnetic Field 140
8.3.2 Magnetic Signals Excited by the Applied Magnetic Field 142
8.4 Conclusions 146
References 147
Part III Evaluation of the Repair Quality of Remanufacturmg Samples by the MMM Technique
9 Characterization of Heat Residual Stress During Repair 151
9.1 Introduction 151
9.2 Preparation of Cladding Coating and Measurement of MMM Signals 153
9.2.1 Specimen Preparation 153
9.2.2 Measurement Method 153
9.2.3 Data Preprocessing 155
9.3 Distribution of MMM Signals near the Heat Affected Zone 156
9.3.1 Magnetic Signals Parallel to the Cladding Coating 156
9.3.2 Magnetic Signals Perpendicular to the Cladding Coating 157
9.3.3 Three-Dimensional Spatial Magnetic Signals 159
节选
Part Ⅰ Introduction to the Metal Magnetic Memory (MMM) Technique Chapter 1 Nondestructive Testing for Remanufacturing 1.1 Motivations Global environmental pollution and resource scarcity have become increasingly serious at present. In addition, many pieces of mechanical equipment have been scrapped from various industries, including the automobile, shipping, aerospace and petroleum industries. Taking China as an example, official statistical data from the China Construction Machinery Association in 2014 indicated that product ownership of engineering machinery reached as high as 7 million, 80% of which exceeded warranty. Moreover, the number of registered and scrapped automobiles reached 33.52 million and 8.58 million, respectively, in 2017, as shown in Fig. 1.1 based on the Chinese automobile market investigation report. It can be predicted that the quantity of automobile scrappage will exceed 18 million in the next few years. In addition, there are still 7.28 million engineering machinery, 8 million high-end machine tools, and 1.2 thousand shield tunneling machines in service at present. All of these machinery will be scrapped or upgraded after reaching lifetime limitations, which has great potential for remanufacturing. Therefore, maximizing the usefulness of waste products has been the key to improving the resource utilization level under this new background. Compared with the products of the traditional manufacturing industry, remanufactured products can reduce costs by 50%, save more than 60% in energy consumption and 70% in materials and reduce emissions by 80%. Taking the first remanufacturing shield tunneling machine in China as an example, the remanufacturing process can save over 20 million yuan, 200 tons of steel, and 260 tons of standard coal while cutting down 700 tons of carbon dioxide. Consequently, remanufacturing exhibits an affinity to the concepts of sustainable production and sustainable society and has been attracting increasing attention worldwide [1]. For example, the USA has the largest industry scale, exceeding 75 billion dollars [2]. Europe enforces recycling and remanufacturing laws for waste automotive parts, and the European Commission lists remanufacturing as the research plan in “Horizon 2020”. Among them, the remanufacturing industry in the UK can create 5 billion pounds of gross national product. The British Lister Petter company can remanufacture 3 thousand disused engines every year. In addition, at least 90% of parts in German automobiles can be reused. In East Asia, Japan exports one-third of its remanufactured products and earns high profits from such practices [3], and China has exerted immense efforts in recent years to promote the sustainable and healthy development of the remanufacturing industry based on the “Made in China 2025” plan. Fig. 1.1 Number variation of automobiles in China: a registered automobiles and b scrapped automobiles Remanufacturing, which is an emerging green technology, is defined as a series of processes that allow end-of-life products and parts to be recommercialized as new products with the same quality, functionality and warranty [4]. The main remanufacturing process consists of disassembling, cleaning, inspecting, repairing and reassembling, as shown in Fig. 1.2. Remanufacturing cores disassembled from used products should be inspected before repair. Cores that satisfy remanufacturability requirements will be restored to their original specifications via a series of repair techniques, including laser cladding, plasma spraying, plasma transferred arc welding and electroplating deposition. During the repair process, a cladding coating is prepared by these surface engineering technologies on the damaged surfaces of the cores so that their performance can be improved and the remanufactured parts can remain in service [5]. Therefore, quality control and evaluation of the remanufacturing process is essential because they are directly related to determining whether the reliability of remanufactured parts can meet the standard for new parts. From the perspective of remanufacturing engineering, inspection plays a key role in assessing the damage degree and repair quality of the cores to ensure that the remanufacturing process proceeds smoothly. Fig. 1.2 Typical remanufacturing process 1.2 Conventional Nondestructive Testing Techniques A nondestructive testing (NDT) technique, which can detect flaws from the interior or surface of a component based on the variations in heat, sound, light, electricity or magnetism attributed to unusual structures and defects, is essential. Compared with traditional destructive testing techniques, NDT has the following advantages: (1) the performance of objects cannot be affected; (2) all the objects can be tested comprehensively rather than sampled in part; and (3) the whole process of manufacturing, service and remanufacturing can be monitored. Therefore,
-
发电厂电气部分
¥34.6¥58.0 -
植物进化的故事
¥19.9¥59.0 -
赶往火星:红色星球定居计划
¥44.5¥58.0 -
数控车工
¥5.9¥11.5 -
低空无人机集群反制技术
¥82.6¥118.0 -
数据驱动的剩余寿命预测与维护决策技术
¥63.4¥79.0 -
手术机器人导航与控制
¥127.4¥169.8 -
射频干扰袖珍手册
¥18.4¥29.0 -
汽车车身构造与修复
¥30.7¥45.0 -
群目标分辨雷达初速测量技术
¥42.4¥69.0 -
秸秆挤压膨化技术及膨化腔流道仿真研究
¥40.6¥55.0 -
NVH前沿科技与工程应用
¥109.7¥159.0 -
电力系统分析
¥23.8¥38.0 -
继电保护原理
¥30.4¥49.0 -
不确定条件下装备剩余寿命预测方法及应用
¥60.4¥99.0 -
船舶分段装配
¥58.6¥80.0 -
基于深度学习的复杂退化系统剩余寿命智能预测技术
¥54.4¥89.0 -
火星探测器轨道动力学与控制
¥59.8¥98.0 -
美军联合作战弹药保障
¥35.8¥58.0 -
工程造价全过程管理系列丛书 工程结算与决算 第2版
¥37.4¥56.0