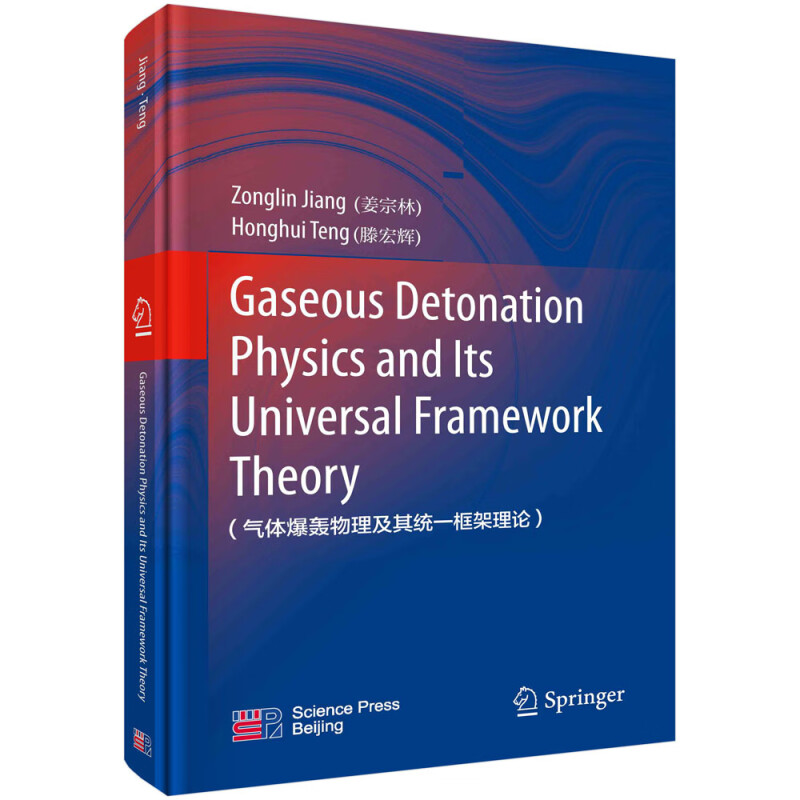
包邮气体爆轰物理及其统一框架理论(英文版)

- ISBN:9787030752437
- 装帧:一般胶版纸
- 册数:暂无
- 重量:暂无
- 开本:B5
- 页数:284
- 出版时间:2023-04-01
- 条形码:9787030752437 ; 978-7-03-075243-7
内容简介
本书介绍了气体爆轰物理的研究进展,在此基础上提出了气体爆轰统一框架理论,并应用该框架理论指导了面向工程的爆轰问题研究。爆轰波的研究已经有一百多年历史,但是理论研究滞后,实验研究手段缺乏。借助近年来迅速发展的数值模拟技术,著者1998年从国外回到中国科学院力学研究所之后,带领团队开展了气体爆轰物理的系统研究。本书系统阐述了著者近年来在爆轰物理方面的研究成果,并对其他团队的相关成果进行了总结,在此基础上提出了气体爆轰统一框架理论。该理论被应用在斜爆轰推进和爆轰驱动激波风洞的研究中,推动了工程进步,取得的成果也得到了广泛关注
目录
1 Introduction 1
1.1 Origin and Cognition of Gaseous Detonation 1
1.2 Explosion, Deflagration and Detonation Waves 2
1.3 Methodology of Gaseous Detonation Research 4
1.3.1 Experimental Research 4
1.3.2 Numerical Research 7
1.3.3 Detonation Theory 9
1.4 Critical Physical Phenomena of Gaseous Detonation 11
1.4.1 Detonation Initiation 11
1.4.2 Wave Structure 12
1.4.3 Detonation Quenching 13
1.4.4 Wave Evolution 14
1.4.5 Stability of Detonation Wave 16
1.4.6 Gaseous Detonation Application 17
1.4.7 Motivation of This Book 19
References 20
2 Mathematical Equations and Computational Methods 25
2.1 Fundamental Theories of Gaseous Detonation 25
2.1.1 B asic Equations 26
2.1.2 Rayleigh Lines and Hugoniot Curves 27
2.1.3 Chapman-Jouguet Theory 30
2.1.4 CJ Detonation Speed 33
2.2 Chemical Reaction Models 34
2.2.1 One-Step Irreversible Heat Release Model 36
2.2.2 Two-Step Induction-Reaction Model 36
2.2.3 Detailed Chemical Reaction Model 38
2.3 Computational Fluid Dynamics Methods 40
2.3.1 Governing Equations 40
2.3.2 Computational Methods 43
2.3.3 Acceleration Technologies of Detonation Simulation 46
2.4 Some Typical Simulation Results 47
2.5 Concluding Remarks 54
References 55
3 Classical Theory of Detonation Initiation and Dynamic Parameters 59
3.1 CJ Theory and ZND Model 59
3.2 Deflagration-to-Detonation Transition 70
3.3 Direct Initiation Through Strong Shock 74
3.4 Detonation Initiation Theory 80
3.5 Important Dynamic Parameters 86
3.6 Relation Among Different Dynamic Parameters 90
References 94
4 Unstable Frontal Structures and Propagation Mechanism 95
4.1 Multiwave Detonation Fronts 95
4.2 Structure Evolution from Nonequilibrium State 100
4.3 Reflection and Diffraction of Cellular Detonations 109
4.4 Cylindrical Expansion Detonations 117
4.5 Strongly Unstable Detonations 123
References .
5 Universal Framework for Gaseous Detonation Propagation
and Initiation 135
5.1 Introduction 136
5.2 Mechanisms Underlying Hot Spot Initiation 138
5.3 Chemical Reaction Zone and Its Evolution 146
5.4 Critical Initiation State and Its Characteristics 152
5.5 Equilibrium Propagation State and Its Averaged Features 157
5.5.1 Mechanisms Underlying Detonation Cell Generation 158
5.5.2 Supercritical Detonation 161
5.5.3 Subcritical Detonation 163
5.6 Averaged Cell Size and Half-Cell Law 166
5.6.1 Cylindrically Propagating Detonation 166
5.6.2 Detonation Cell Bifurcation Mechanism 168
5.6.3 Half-Cell Rule of Detonation Propagation 170
5.7 Detonation Cell Correlation with Ignition Delay Time 172
5.7.1 Ignition Delay Time 172
5.7.2 Cell Size Correlation 175
5.7.3 Detonation Reaction Modeling 177
5.8 Applications of the Universal Framework 180
5.9 Remarks on the Universal Framework 184
References 184
6 Structures and Instability of Oblique Detonations 187
6.1 Conservation Laws and Polar Analysis of Oblique Detonations 187
6.2 Wave Structure of Initiation Region 191
6.3 Multiwave Structures on an Unstable Surface 198
6.4 Oblique Detonation Waves in Nonideal Inflow Conditions 208
6.5 Effects of Rear Expansion Waves Derived from Finite-Length Wedges 214
6.6 Effects of Blunt Body on Initiation 220
6.7 Remarks on Oblique Detonations 225
References 226
7 Engineering Application of Gaseous Detonations 229
7.1 Thermal Analysis of Detonation-Based Combustion Process 229
7.1.1 Thermal Cycle Efficiency for Isobaric Cycles 230
7.1.2 Thermal Cycle Efficiency for Isochoric Cycle 233
7.1.3 Thermal Cycle Efficiency for Detonation Cycle 236
7.1.4 Comparison of Thermal Cycle Efficiency for Isochoric, Isobaric and Detonative Engines 240
7.2 Propulsion Technology Based on Detonation Combustion 243
7.2.1 Pulse Detonation Propulsion Concept 245
1.2.2 Oblique Detonation Propulsion Concept 247
7.2.3 Rotating Detonation Propulsion Concept 252
7.2.4 Key Technologies for Detonation Engines 256
7.3 Shock Tunnel Driven by Gaseous Detonations 258
7.3.1 Principles of Detonation-Driving Shock Tube/Tunnel 260
7.3.2 Development of Detonation-Driving Shock Tunnel 262
7.3.3 Transient Testing Techniques in High-Enthalpy Shock Tunnels 267
References 270
节选
Chapter 1 Introduction Detonation is one of the most distinguished phenomena in gas dynamics, and it is characterized by nonlinearity, strong discontinuity and the tight coupling of chemical reaction and shock wave. A detonation wave is a supersonic combustion wave across which the pressure and temperature of combustion products increase sharply. Since the phenomenon of detonation was first observed scientifically over one hundred years ago, there have been numerous studies on detonations, from fundamental physics to application technologies, such as severe explosion prevention, supernovas in astrophysics, and for military purposes. Detonation has been “applied” for military and mining and observed in nature but was not well understood. In the last two decades, detonation applications in aerospace propulsion and high-enthalpy shock tunnels have attracted worldwide attention. In this chapter, the origin and current understanding of gaseous detonation are briefly reviewed at first, and then, a description of the critical issues in detonation research is given, which will be further discussed in the following chapters of this book. 1.1 Origin and Cognition of Gaseous Detonation Detonation is an intense supersonic combustion phenomenon with extreme speed that occurs in nature and influences the development of human civilization. Early observation and research on detonation are derived from accidental explosions in coal mines, which were also observed in chemical plants. The terrible destruction cannot be explained by fire or the usual explosion, thus attracting attention from scientific researchers. In coal mines, methane leakage is unavoidable and some dust is produced. Their mixing with air creates a circumstance of detonation initiation, which could be triggered by casual ignition, such as a spark or mechanical impact. The initiated detonation propagates with a high speed led by a strong shock, which could compress and ignite the combustible gases. For hydrogen-fueled detonation,the speed could reach as high as 2000 m/s. Early researchers paid attention to how to prevent the hazardous effects of detonation, such as suppression of detonation initiation and weakening its destructive power, which are important to coal mine production and chemical plant design. Detonation is essentially a chemical explosion that has a long research history. Abel [1] measured the detonation wave speed in a pioneering study in 1869. Berth-elot and Vieille from France [2] in 1881 performed a systematic study of the detonation wave speed for various reactants, including H2, C2H2, and C2H4 fuels, and the evidence of detonation existence has been proven substantially. Another critical experiment was performed by Mallard and Le Chatelier in 1883 [3], which confirmed that both deflagration and detonation could be ignited in one combustible mixture and recorded the deflagration-to-detonation transition (DDT) process. As further shown, the leading shock of the detonation wave in front of the heat release zone compresses the gas and induces combustion, whose mechanism is essentially different from that of deflagration. These early works provide a primary concept of detonation and its features. In the 1990s, detonation phenomena were studied worldwide and became an interdisciplinary subject. There are a number of monographs on detonation from various viewpoints [4—9], reviewing the theoretical, numerical and experimental progress at different periods. From the viewpoint of combustion, the leading shock provides a circumstance of high pressure and improves a fast and efficient combustion mode, in turn improving the thermodynamic cycle efficiency. Moreover, under this pressure-gain combustion, more energy is converted to mechanical energy than with constant-pressure combustion given the same initial conditions. Therefore, advanced engines based on detonation have great potential to be superior to current engines based on deflagration, leading to the concept of pulse detonation engines, rotating detonation engines and oblique detonation engines. In addition to the engines, detonation could also be used in shock tunnels, especially high-enthalpy shock tunnels. Two driving patterns were proposed: backward-driven and forward-driven patterns, which increase the total pressure of the shock tunnel to different extents [10-12]. We believe that more detonation applications will be proposed in the future and hope that gaseous detonation research will continuously promote engineering development. 1.2 Explosion, Deflagration and Detonation Waves Although detonation has a clear definition and unique features, some researchers in related communities may confuse detonation with two other waves: explosion waves and deflagration waves. Therefore, we first distinguish these different concepts in this subsection. The explosion results in a strong shock, which compresses the surrounding medium and changes the gas dynamic p
-
宇宙与人
¥13.0¥35.0 -
新太阳系
¥13.2¥35.0 -
物理学之美-插图珍藏版
¥23.1¥69.0 -
世纪幽灵-走近量子纠缠
¥11.8¥28.0 -
技术史入门
¥21.1¥48.0 -
假设的世界:一切不能想当然
¥19.1¥45.0 -
东京文艺地图(九品)
¥14.2¥38.0 -
数学的魅力;初等数学概念演绎
¥12.5¥22.0 -
118个数学竞赛不等式
¥45.5¥78.0 -
刘薰宇的数学三书:原来数学可以这样学全3册
¥35.2¥118.0 -
昆虫的生存之道
¥20.1¥38.0 -
沉积岩与沉积相
¥28.6¥41.0 -
量子宇宙:一切可能发生的正在发生
¥16.1¥32.8 -
科学与现代性:整体科学理论
¥18.2¥33.0 -
20世纪中国科学口述史:涂光炽回忆与回忆涂光炽
¥24.2¥46.5 -
运筹学原理与方法
¥24.0¥72.0 -
从一到无穷大
¥25.9¥46.0 -
普林斯顿微积分读本-(修订版)
¥69.3¥99.0 -
空间行为的地理学
¥44.6¥89.0 -
古文诗词中的地球与环境事件
¥12.0¥28.0